8.2. Work#
Do you appreciate that the clay drop is, well different, but really the same as Herman and Blanche’s bruised hands? They’re both an impact and embedding of a projectile with a flexible target.
Imagine that a ball of a given velocity, \(v_1\) is thrown at model of your hands, which are very clever hands since they can determine the depth of the resulting bruise:
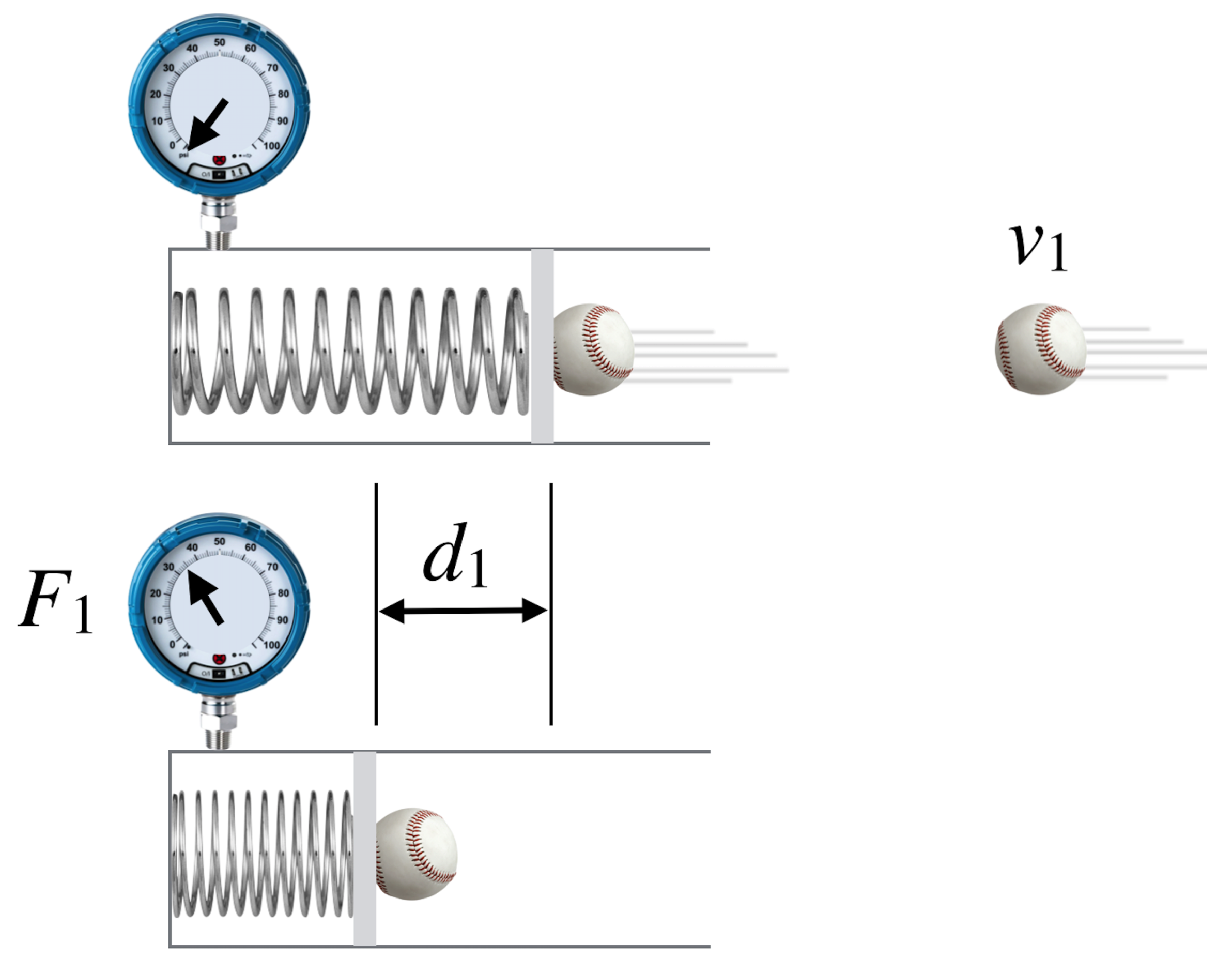
What happens in the inside, so to speak? Your hands are flexible and squish-able (the spring) and will compress with the ball’s impact but will also apply a varying, but here an average force back at the ball, slowing it to a stop. That’s represented by the \(F_1\) while the depth through which that force pushes back is the length \(d_1\) in the diagram. It turns out that the product of these two latter quantities is a very useful item.
The fancy way to speak about this \(F_1 \times d_1\) quantity is in terms of “work” which means something very specific in physics. Work is in fact that product of (force \(\times\) the distance through which the average force acts), \(W=F\Delta x\).
This is similar to the way that Impulse is the product of (force \(\times\) the time through which the force acts). Sometimes the symbol \(J\) stands for impulse, and so we’ll name it in order to stand in comparison to work: \(J=F\Delta t\). And here’s the connection to our concern: that quantity \(F\Delta x\) is equal to the change in the kinetic energy of the object, in this case, the baseball:
which looks a lot like
This apparent partnership between time and space is related to the partnership between energy and momentum, as we’ll see a bit later.
Work is equal to the change in kinetic energy – a force applied through a distance – in a similar way that Impulse is equal to the change in momentum – a force applied during a time interval.
Let’s look deeper.
Here’s a derivation of the relationship between work and the change of kinetic energy
Here’s a different take on what we’ve done so far:
Please study Example 1:
Please answer Question 1 for points:
Please answer Question 2 for points:
Please study Example 2:
8.2.1. Now Let’s Explain “Damage”#
I began with the idea of “damage” and now it’s time to explain myself. A rearrangement of the internal “parts” of any colliding object comes from the kinetic energy of the colliding objects. Take Blanche’s hands. Her hand-parts are her skin, facia, muscles, blood, tendons, ligaments, and bones. When the ball strikes her skin, it transfers momentum to all of those parts along the ball’s direction, sure. But it does that by distributing momentum among the elements of her hand…pieces of which move internally causing them to gain speed very quickly, for a very short distance. That is, pieces of her hand do work on other pieces of her hand and the kinetic energy of those pieces changes—often at the molecular level. Some of that momentum transfer is in the direction of the ball—once her palm is squished to its limit, then the rest of her moves that direction because her wrist and elbow resist almost rigidly. She balances momentum along that ball’s direction by moving her whole body a bit. But some of the momentum transfer is not in the original direction, because her hand is made of…parts.
Since kinetic energy is not a vector, there will be some compression and tissue tear (which requires Work to accomplish) in all directions, say towards her thumb which will be balanced by some other compression and tissue tear in the opposite direction, say opposite, towards her little finger, and so on. In each little disruption, momentum is balanced (thumb-finger), but the motions are in all directions. So very quickly, the original ball’s velocity is given up to a) Blanche as a whole along the ball’s trajectory—which we say from the first graph is very little—and b) the individual pieces in all directions which make up Blanche’s hand. If you could capture and measure all of the speeds of the pieces of her hand, you could get closer to the original kinetic energy balance that seems so out of whack when you deal only with the whole Blanche and the whole ball. These bits of motion collectively make up “internal energy” of a system. The end result, after the big bits of her hand have settled down comes from Mr Joule: heat. Molecules, crystals, tissues, etc are still vibrating and rotating and translating…these motions even in solids are the definition of heat.
Let’s keep track of all of the little bits of momentum and energy transfer as the ball leaves the pitcher’s hand and settles into the bruise that it makes on Blanche’s hand.
On its way, the air in front of the ball is compressed, which means that the air molecules are accelerated and move faster than before…that is, the air is heated and according to Joule’s work, that takes away some of the ball’s kinetic energy.
The ball is spinning and the drag on the air likewise locally compresses and rarefies the air—the seams on a ball dig into the air and make the ball curve and drop, but also result in turbulence along the path and, you maybe guessed it, heat up the air along the way.
You can hear a ball go by. If you’ve ever nearly been beaned in the head by a fastball, you know this. That compression above actually propagates away from the ball’s trajectory making the air create a compressional wave which eventually has hit your ear drum. That wave does Work on your eardrum and internally—molecularly—makes it warm. More heat-energy transferred away from the ball.
When the ball hits Blanche’s hand it does all of the Work that is described above but eventually the moving tissues become heat—her hand will feel warm because blood has arrived to repair the damage.
You’ll hear the ball hit her hand! Again, the vibrations of the ball and her hand will create compressional waves that will leave the collision at the speed of sound and warm up all of the ear drums of every spectator and player. More heat.
Finally, in parallel with the bits of her hand compressing, twisting, bending, and warming…the ball also will distorts, compresses, and vibrates—and gets warm—and releases that tension and adds to the sound.
In the end, all of the lost kinetic energy becomes heat, whether in a completely inelastic collision like catching a baseball, or only a “regular” inelastic collision. Think of the sound that even very hard billiard balls make when they collide—they’re very briefly compressing and vibrating and that excites the air and you hear it as your eardrums warm up. Have you ever felt a racquetball after a long volley? It’s warm.
This is why speeding bullets can do so much damage. They’re light and they travel fast. A mustket ball from an 18th century dueling pistol is 13.9 grams and travels at about 250 m/s. It’s light and people are heavy and so the amount of momentum transfer from the ball to the victim is small, but from the figure in the first deeper look (the mustket ball is c) the amount of kinetic energy lost is enormous: \(\delta =99.994\%\). All doing tragic damage. A modern bullet can travel twice or more that speed and further breaks up and twists on contact further increasing the damage.
Understanding and modeling inelastic — real-macroscopic-life — collisions can be very complicated.
Fortunately for us, QS&BB is all about individual elementary particle collisions and the distinguishing feature of elementary particles is: no parts. So without parts, our collisions are completely elastic, essentially perfectly rigid: ideal billiard balls, then electrons, protons, photons, and neutrons.
Let’s clear up a confusion from the last lesson.