15.3. Moving Through The Ether#
A naval analogy, as described by Michelson to his children.
Suppose you and your friend are standing by a river and ready to race. The river is still as shown in the figure and you each plan to pilot your motor boat a distance \(L\), starting at point \(A\) and ending at point \(A\). One of you goes across the river (\(AB\) to \(BA\)), and the other of you to the right and then left (\(AC\) to \(CA\)). Who wins if both boats can move through the water at the same speed?
Pencils Out! 🖋 📓
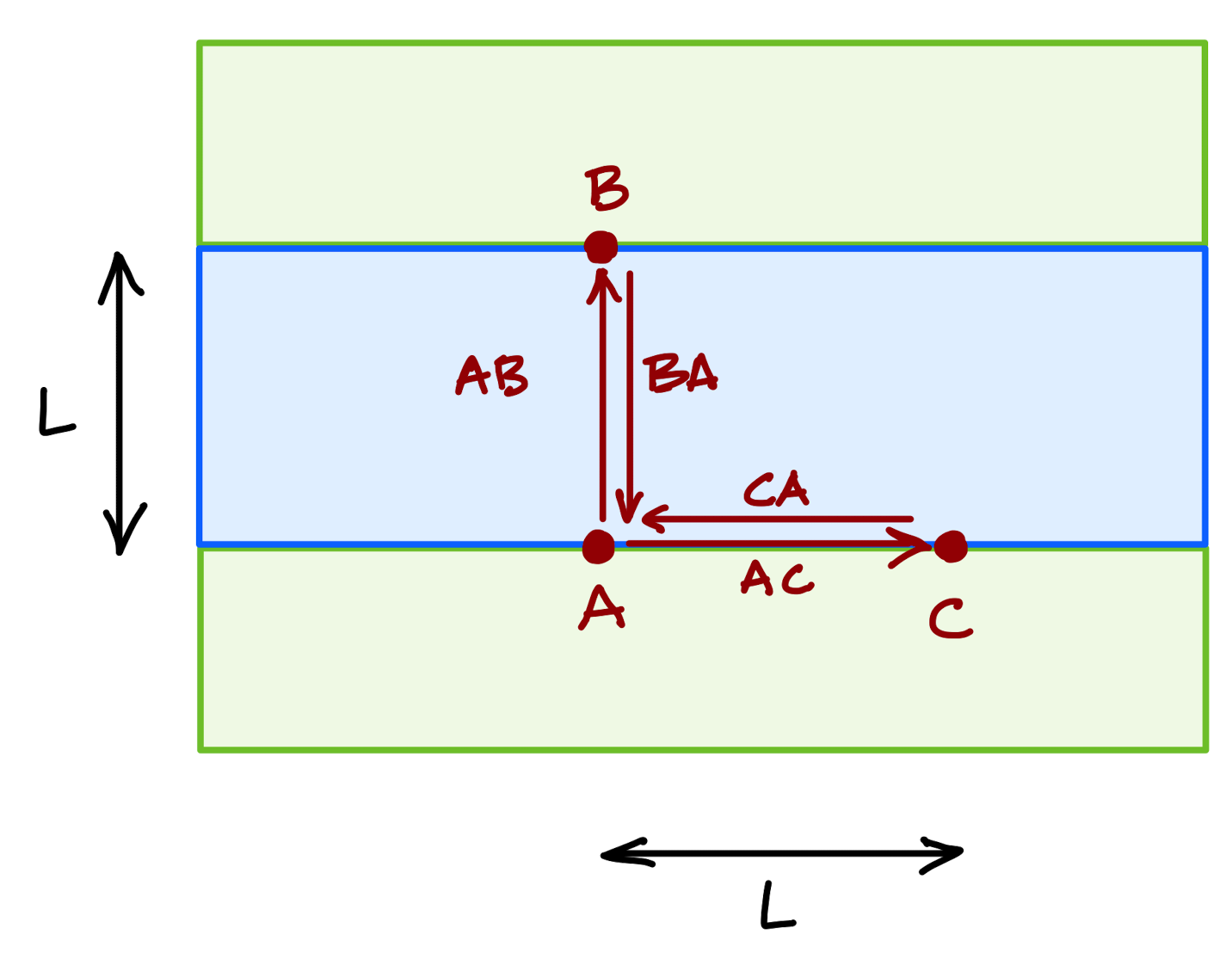
Obviously, both of you are traveling at the same speed, over the same distance and if the water is perfectly still (no current), your race from \(AB\), \(BA\) compared with \(AC\), \(CA\) would result in a tie. That’s too easy.
Now suppose that the river has a strong current to the left. I take the right-left trip and in the first leg, \(AC\) I must go against the current while the return \(CA\) is helped by the current. By contrast, you take the trip \(AB\) but you can’t go directly across the river since your boat would be carried downstream, so you must aim your boat to the right at just the right angle to drift to \(B\) as you plow through the water at your regular speed. Then you return has to be the same thing.
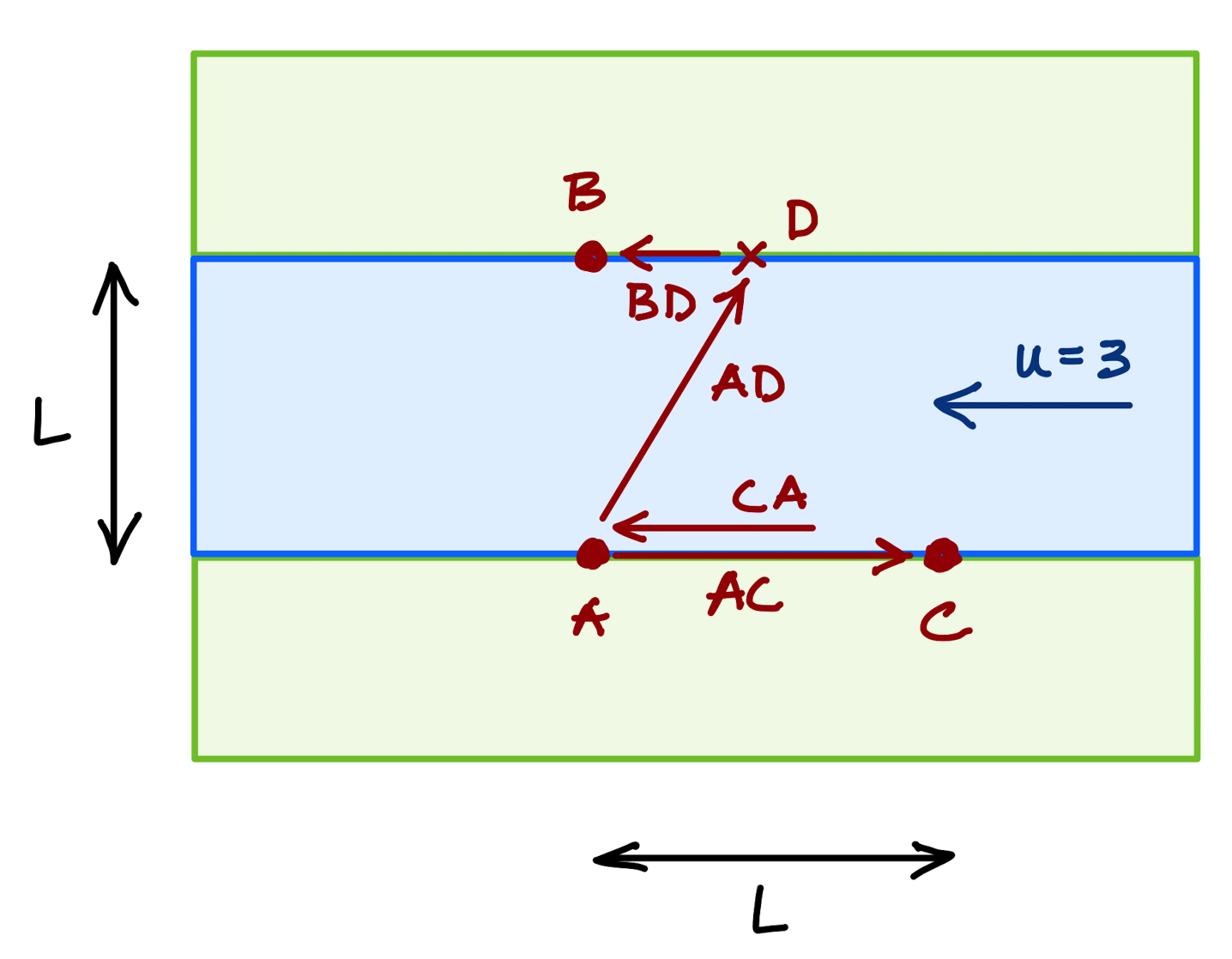
Who wins?
Well, it turns out that the round trip across the river will be quicker than the trip to the right and to the left.
Now suppose we make the following substitutions in our nautical race:
boat\(\to\) light beams
river\(\to\) the ether “wind” as the Earth passes through it
bank\(\to\) the Earth
From the position of the Earth, the ether moves past to the left as the Earth moves in its orbit, here to the right. So on Earth we should detect a constant “ether wind.” Or a current to the left. Here is Michelson’s instrument riding on the Earth and together they move through the fixed ether:
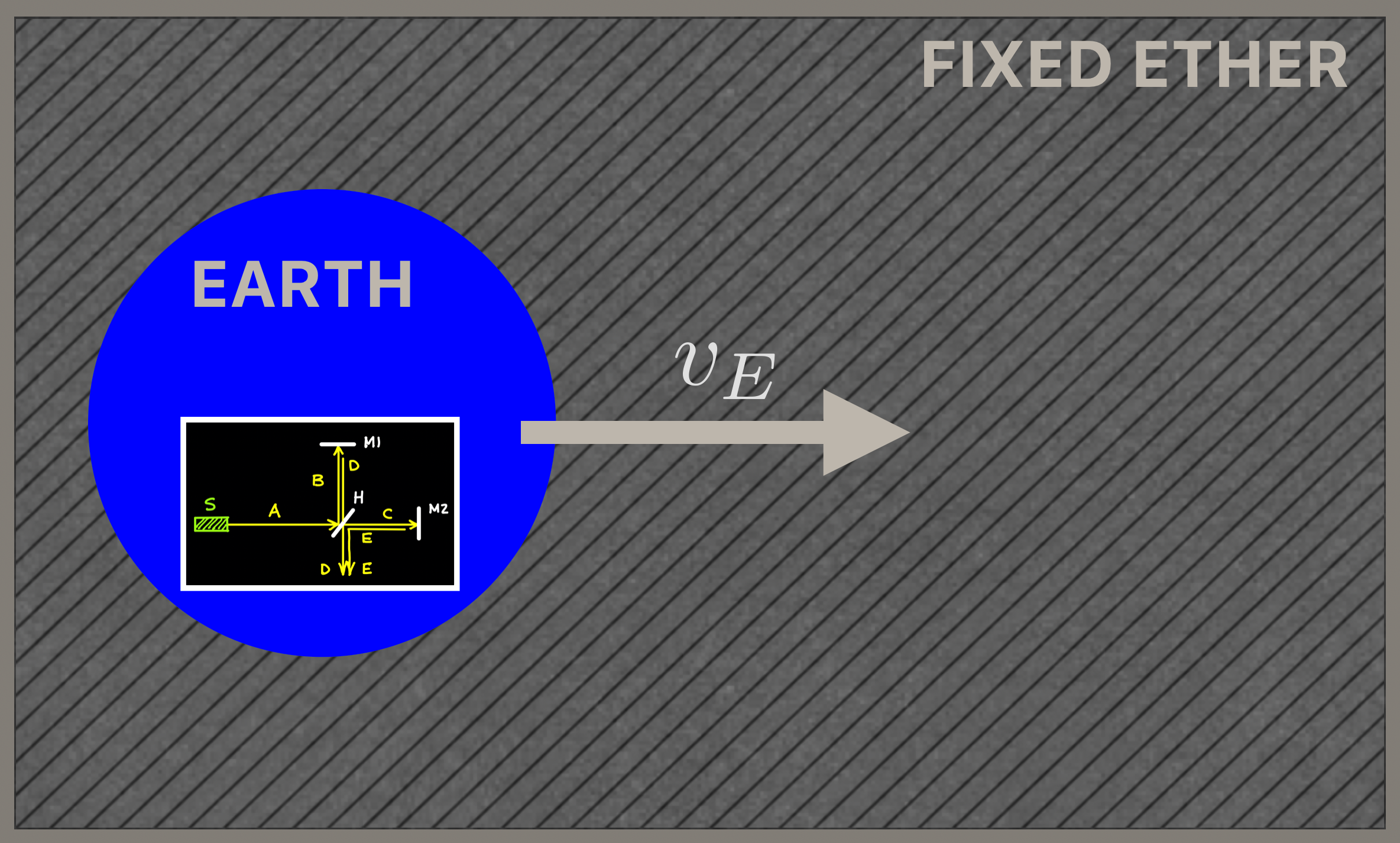
15.3.1. Michelson’s Interferometer#
How did he do this? The instrument he invented and spent his life perfecting is called the Michelson Interferometer and it’s a standard tool in today’s laboratories in optics and even astronomy.
Pencils Out! 🖋 📓
Here’s the idea:
Suppose we have two identical trains that leave the station on identically constructed tracks at precisely the same speeds. Each train has 4 cars, each car is exactly the same length, and the spacing between the cars is exactly the same. Our little trip is kind of boring. It consists of the following set of loops created so that the tracks and so the trains pass one another at the top. We’re at point \(O\) and there’s a light source on the other side of the trains from us.
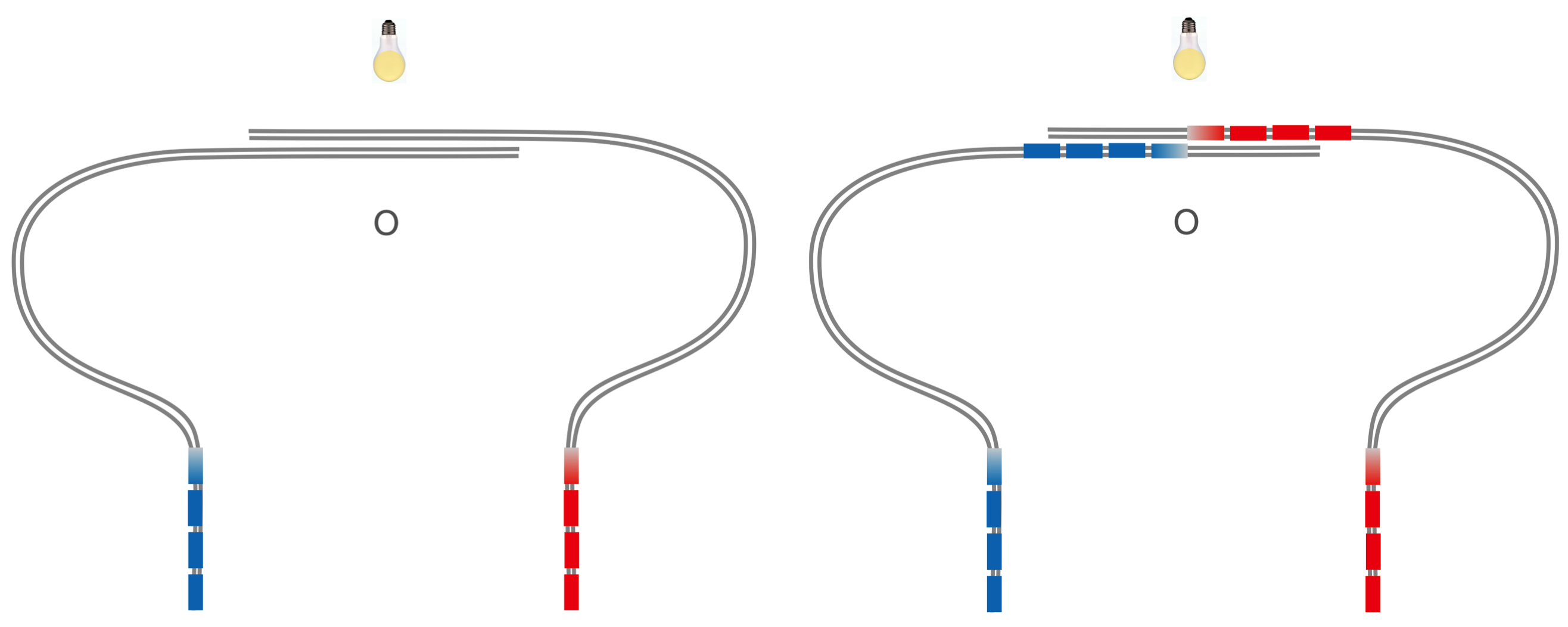
The right figure shows when they are just about to pass by one another in they synchronized trip. We stand at point O and we'll watch for light from the bulb on the other side of the tracks.
On the left, this figure shows the trains just starting out on their trips. On the right they have reached the mid-point and are just beginning to pass one another. Notice that the fronts line up with blue going right and red going left.
Let’s look at successive times. From A to B to C, we have snapshots of the train positions from early to later times. In each case, the gaps between the cars line up and from O we can see the light.
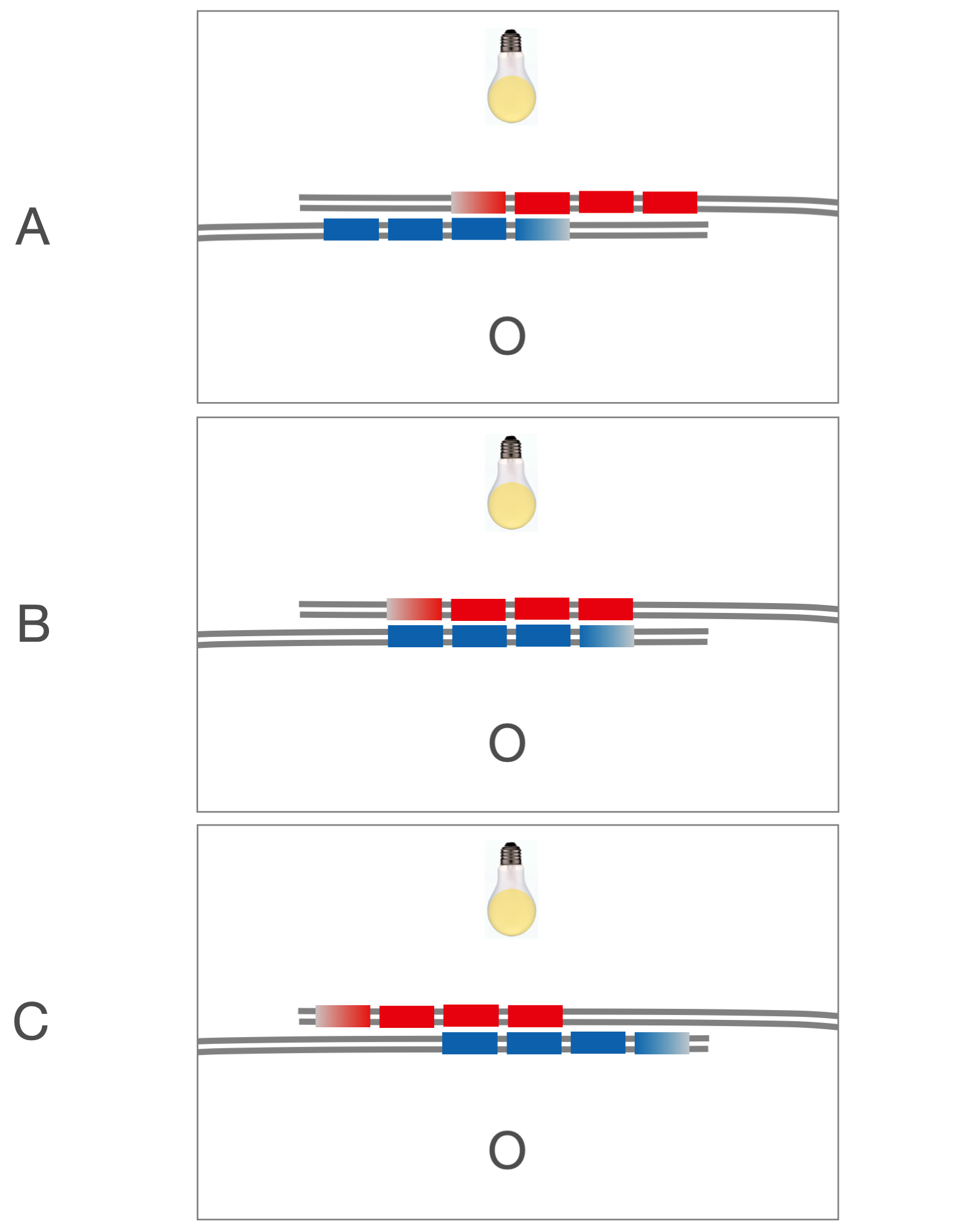
Now, lets’ mess with them and make the right-hand track just a tiny big longer than the left-hand track. Same trains. Same speeds. Now the left-hand blue train reaches \(A\) slightly before the right-hand red train, right? So the gaps are out of phase and you don’t see the light except only occasionally. From top to bottom, we can see that the gaps never will line up.
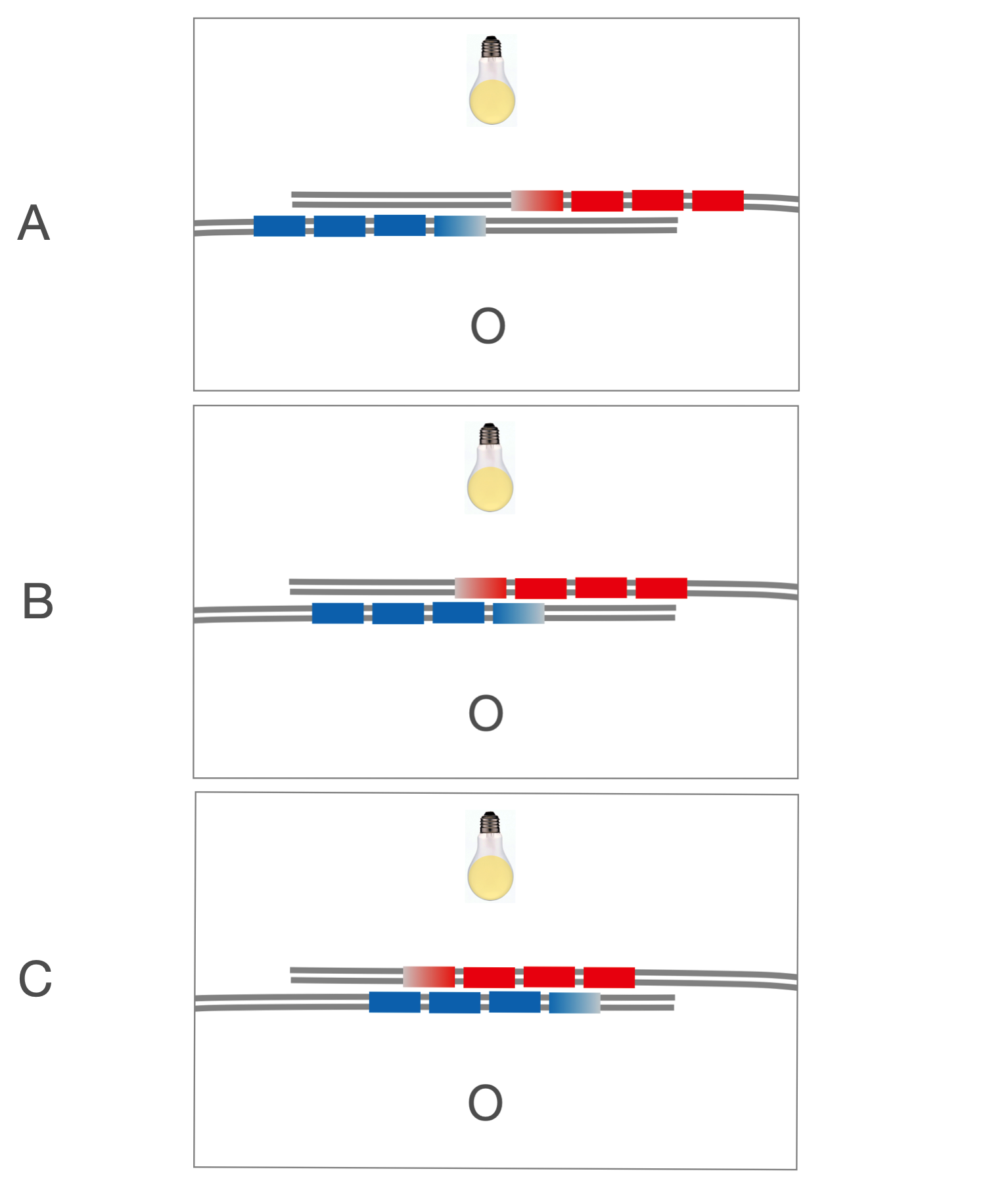
Now, we’ve messed with the synchronization and the two cars from red and blue are not in time with one another and the likelihood of seeing the light? Very small. It’s blocked.
Suppose (and I don’t know how to build this), the right -hand track is not getting longer, but moving away toward the right, away the other one by stretching the track. Can you see that this too would make the gaps not line up, except only occasionally?
That’s the principle behind the Michelson Interferometer. Instead of trains, he used light. Instead of gaps, the peaks and valley’s of the light waves either would be in phase and get bigger in synch – like seeing through the gaps between the cars – or they would not and interfere and you’d see the peaks diminished or even gone.
Michelson invented and built (on Earth!) a first version of an instrument that sent a beam of light in one direction, like \(AC\) on the river and at the same time a second beam of light in a perpendicular direction, like \(AB\) on the river. Then with mirrors he brought them together back at \(A\) to see who wins. What determines winning?
Well, light is a wave and when two waves encounter one another, they mix and interfere. Where the two waves are in-synch, the result is a new wave that’s big at the position of the original common peaks. Where they’re out of synch? Well, then you get a quiet result–no wave (think of your noise-cancelling headphones). If they’re somewhere in-between, then there’s a peak, but it’s not where the peak is for either of the two initial waves. So calibrate your instrument carefully for both beams in-synch and then turn it loose on the ether and see what happens.
Here we have two waves that overlap and because they are completely in phase, their overlap (the orange) is at the same place as the two initial peaks.
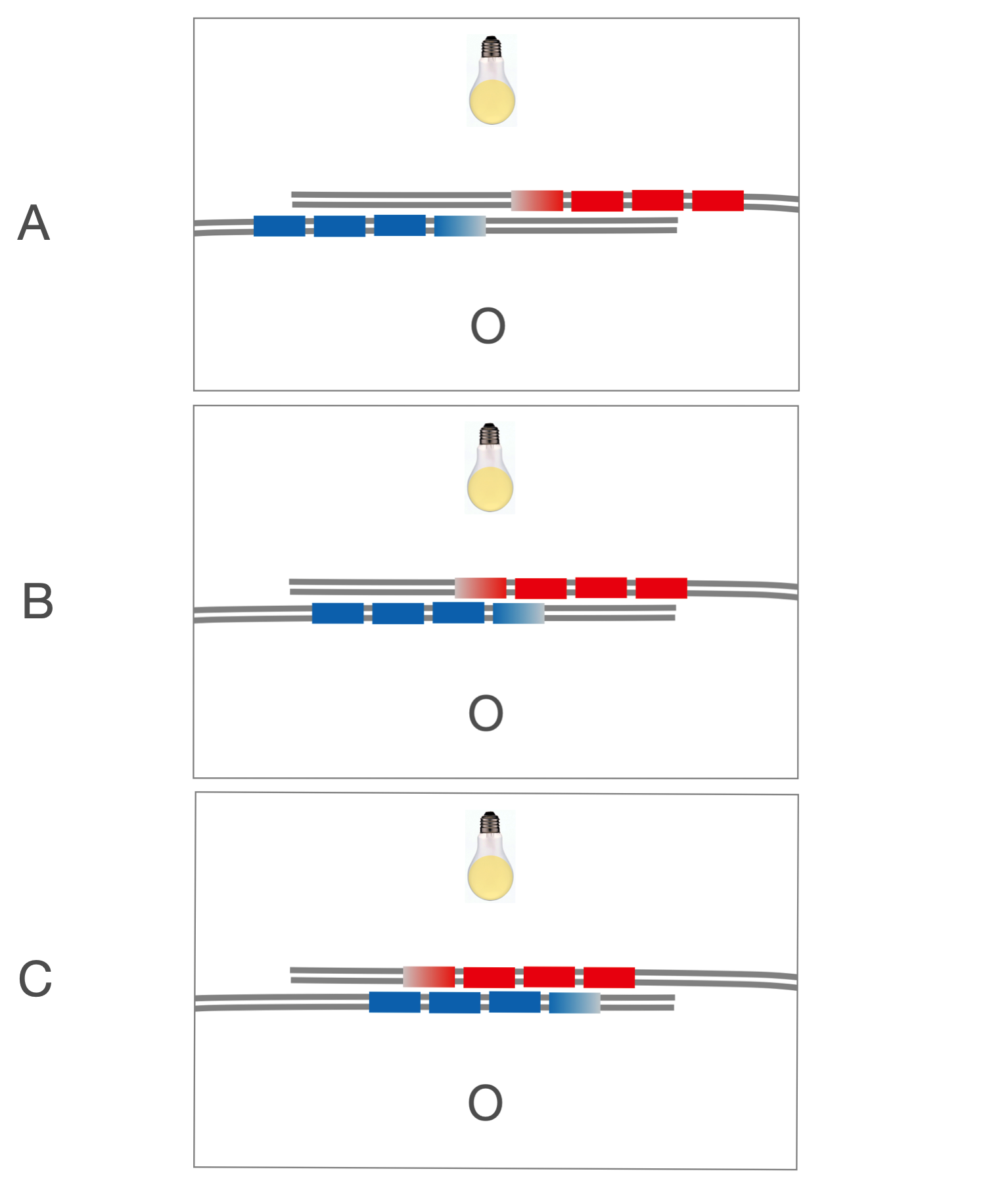
Suppose (and I don’t know how to build this), the right -hand track is not getting longer, but moving away toward the right, away the other one by stretching the track. Can you see that this too would make the gaps not line up, except only occasionally?
That’s the principle behind the Michelson Interferometer. Instead of trains, he used light. Instead of gaps, the peaks and valley’s of the light waves either would be in phase and get bigger in synch – like seeing through the gaps between the cars – or they would not and interfere and you’d see the peaks diminished or even gone.
Michelson invented and built (on Earth!) a first version of an instrument that sent a beam of light in one direction, like \(AC\) on the river and at the same time a second beam of light in a perpendicular direction, like \(AB\) on the river. Then with mirrors he brought them together back at \(A\) to see who wins. What determines winning?
Well, light is a wave and when two waves encounter one another, they mix and interfere. Where the two waves are in-synch, the result is a new wave that’s big at the position of the original common peaks. Where they’re out of synch? Well, then you get a quiet result–no wave (think of your noise-cancelling headphones). If they’re somewhere in-between, then there’s a peak, but it’s not where the peak is for either of the two initial waves. So calibrate your instrument carefully for both beams in-synch and then turn it loose on the ether and see what happens.
Here we have two waves that overlap and because they are completely in phase, their overlap (the orange) is at the same place as the two initial peaks.
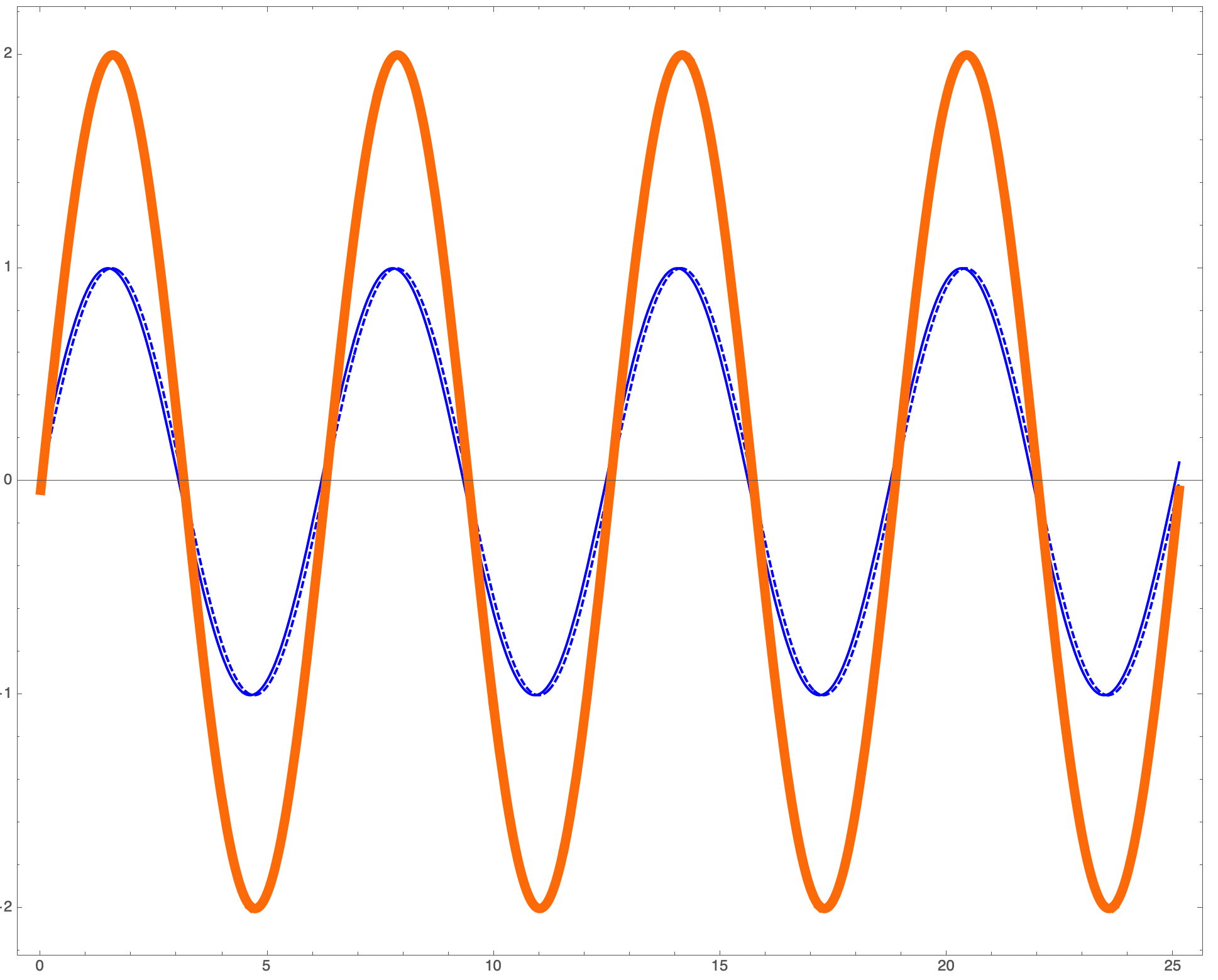
Now let’s suppose that the two initial waves are out of time with one another, as if one arrived before the other.
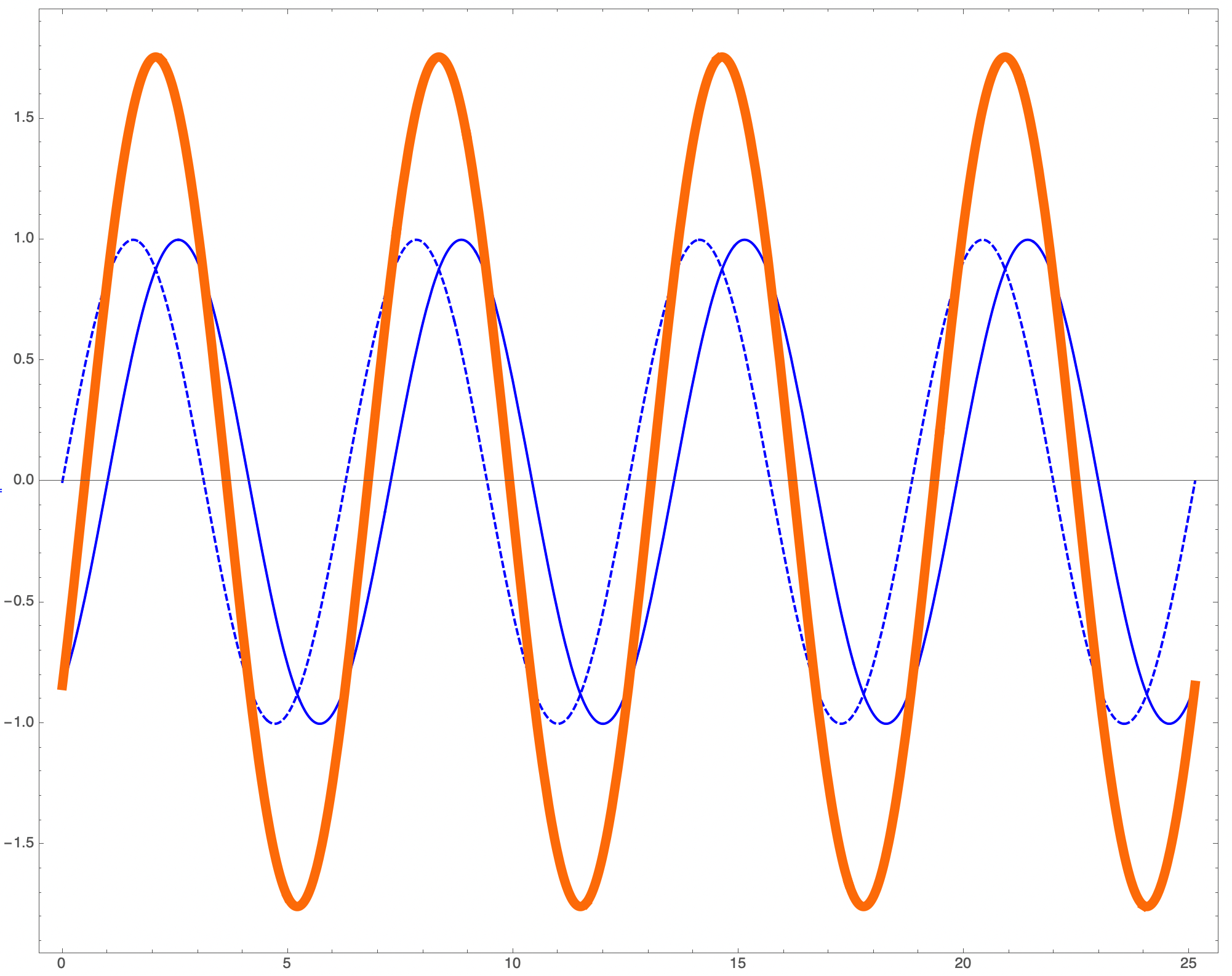
Notice that the two orange curves in the two figures are slightly offset. Here’s a superposition of the two figures for the first cycle. I’ve stared the dashed initial curve for the out-of-phase scenario at the same point as the common, in-phase scenario.
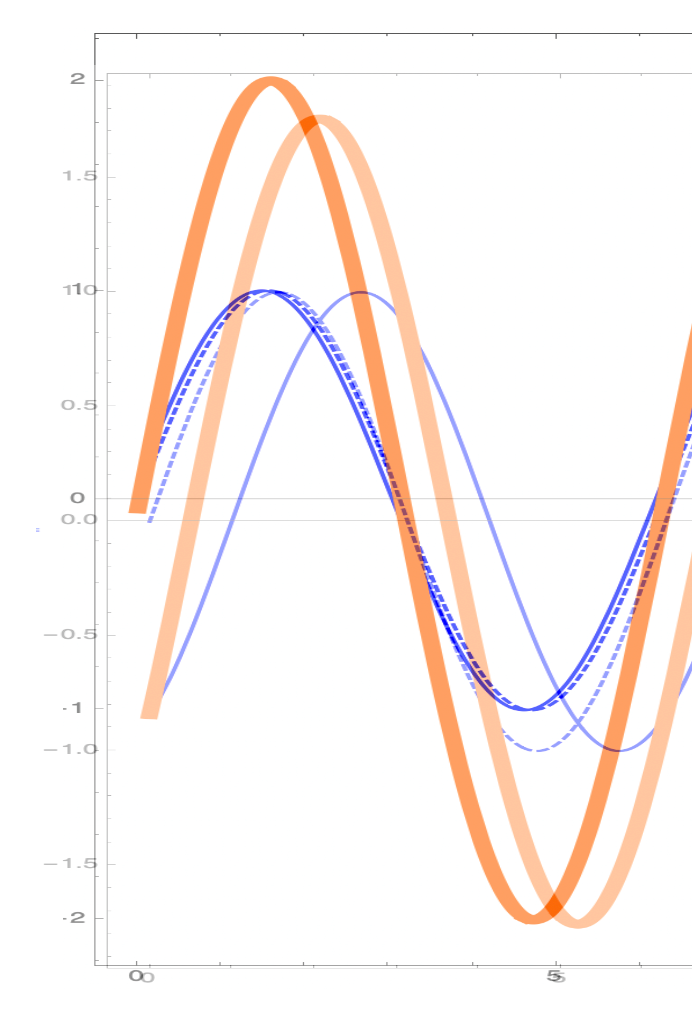
Michelson expected to see the “fringe shift” of the light-orange curve, lagging the in-phase curve as one leg of his interferometer went against the ether or along with the ether.
15.3.2. With The Phone Guy’s Help#
With financial assistance from Alexander Graham Bell and a German optical company, in 1881 Michelson built an exquisitely precise interferometer which combined waves in exactly this way.
Here’s a cartoon illustrating how this worked, the device that’s riding on the moving Earth:
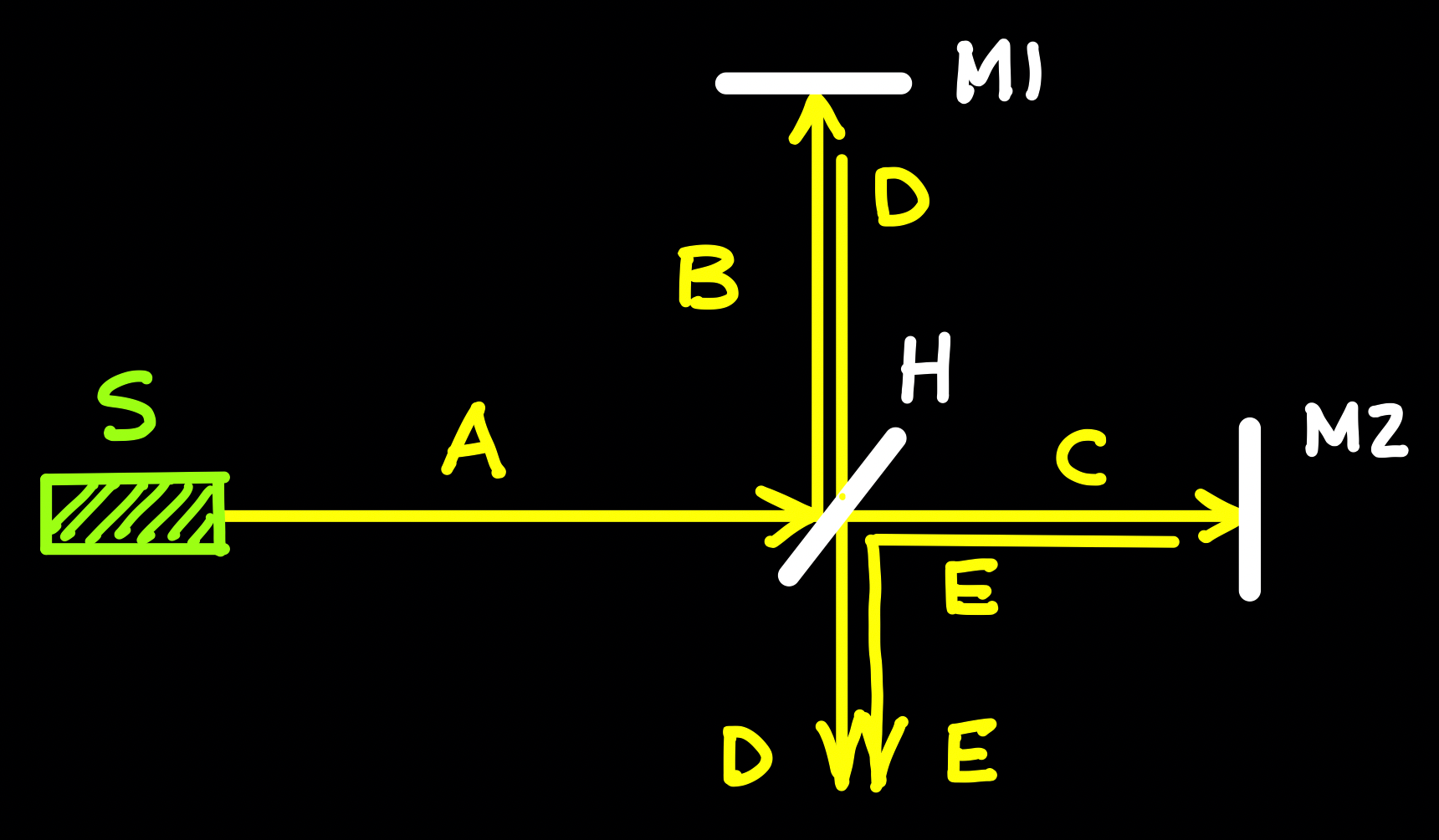
His first prototype had arms about a meter long.
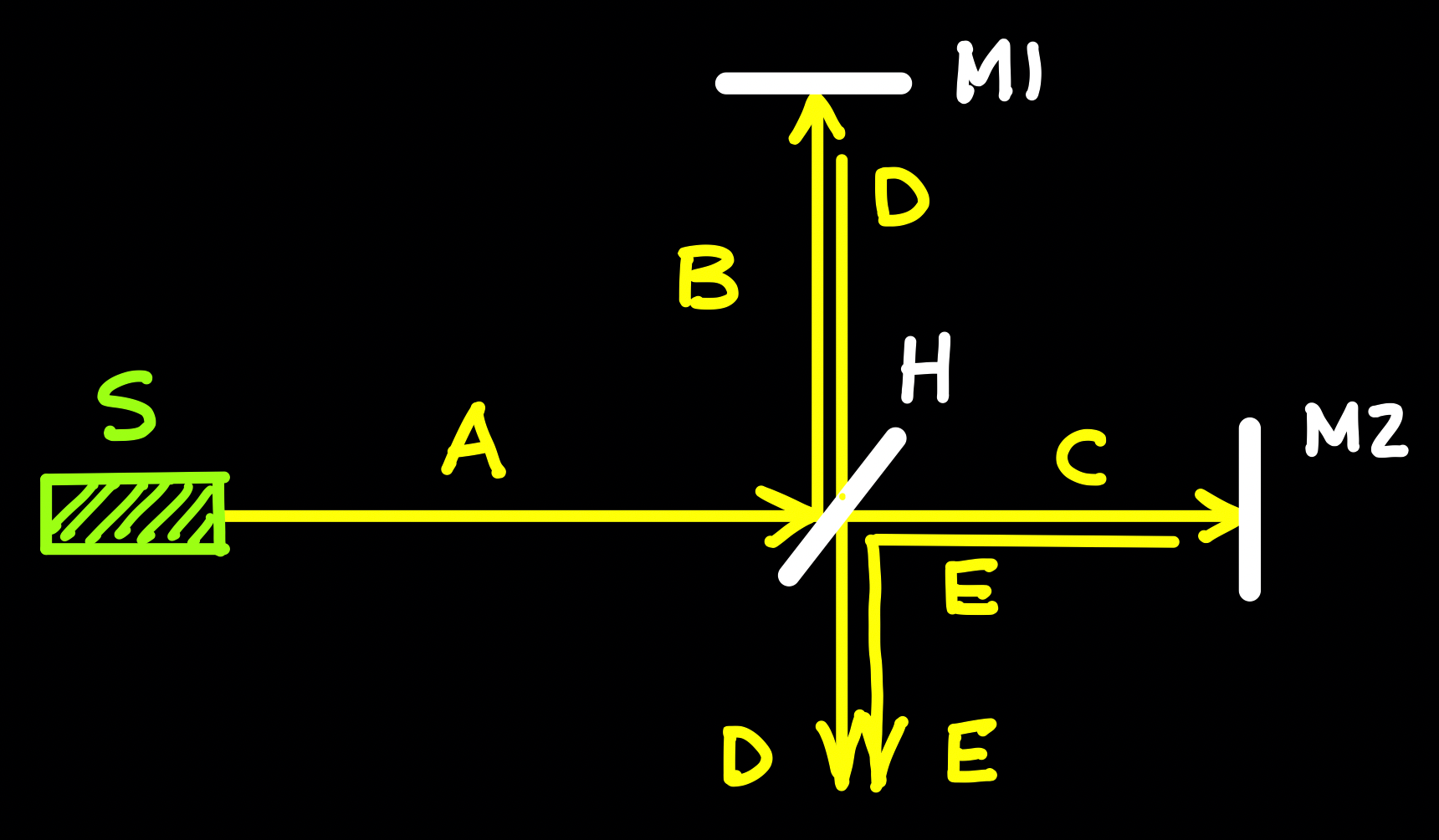
He knew how accurate his device needed to be because the effect of the two light beams would be of the order of
This required a ridiculous level of precision, which Maxwell had calculated and decided it to be impossible. His first instrument was not up to it as even traffic outside of the lab building was sufficiently disruptive to ruin his measurements. He subsequently moved it to a basement in a lab at rural Potsdam in suburban Berlin where the measurement was better, but unsatisfying. After more than six months of painstaking work, he published his results and wrote to his benefactor:
Heidelberg, Baden, Germany
April 17th, 1881
My dear Mr. Bell,
The experiments concerning the relative motion of the earth with respect to the ether have just been brought to a successful termination. The result was however negative…
At this season of the year the supposed motion of the solar system coincides approximately with the motion of the earth around the sun, so that the effect to be oserve [observed] was at its maximum, and accordingly if the ether were at rest, the motion of the earth through it should produce a displacement of the interference fringes, of at least one tenth the distance between the fringes; a quantity easily measurable. The actual displacement was about one one hundredth, and this, assignable to the errors of experiment.
Thus the question is solved in the negative, showing that the ether in the vicinity of the earth is moving with the earth; a result in direct variance with the generally received theory of aberration…
N.B. Thanks for your pamphlet on the photophone.
The speed of the ether relative to the Earth is zero. It was the first failure that Michelson had endured in his so-far, distinguished career as the young King of Optics.